Shallow Foundation Design and Its Advantages
Post provided by: Wafflemat structural systems
Author: Anna Leija-Olveda, MSCE, VP of Engineering, Wafflemat
An Innovative Approach for Enhanced Structural Performance
Introduction
Shallow, slab on grade foundation design is a popular choice for low to mid-rise buildings due to its simplicity, cost-effectiveness, and ease of construction. In this type of foundation, a concrete slab is directly poured on a prepared soil surface, providing a sturdy base for the structure. Common shallow foundation designs consist of traditional ribbed slabs, uniform thickness foundations, pier and beams with a crawl space, spread footings and wall footings. The International Building Code references design guides for the use of shallow foundation design (Figure 1).

Key Objectives
Key aspects and considerations for shallow, slab on grade foundation design:
1. Load Demand: As an engineer, the first step is to determine the load demands of the structure. This includes considering the dead loads (weight of the building components), live loads (occupants, furniture, equipment, etc.) and environmental loads (rain, seismic, wind, etc). The foundation must be designed to safely transfer these loads to the underlying soil.
2. Soil Parameters: Understanding the soil conditions is crucial for designing a successful slab on grade foundation. Conducting a geotechnical investigation helps determine the soil’s bearing capacity, settlement characteristics, and potential for expansive or collapsible soils. These factors directly impact the foundation’s design and performance.
3. Slab Thickness: The slab thickness depends on the load demands and the soil’s bearing capacity. A thicker slab is required to distribute heavier loads, while a thinner slab may be suitable for lighter loads. Proper reinforcement, like steel bars or post tensioned strands, can enhance the slab’s strength and prevent cracking.
4. Joint Placement: Control joints and expansion joints are essential to control cracking caused by temperature changes and moisture fluctuations. Properly placed joints ensure that any potential cracks occur in controlled locations, reducing the risk of structural damage.
5. Insulation: In regions with extreme temperatures, insulation is often added beneath the slab to improve energy efficiency and prevent frost heave during colder months.
6. Vapor Barrier: A vapor barrier is placed beneath the slab to prevent moisture from rising into the building, which can cause various issues such as mold growth and flooring damage.
7. Soil Compaction: Proper compaction of the soil before placing the slab is critical to avoid settlement issues. Compaction ensures the soil can adequately support the weight of the building.
8. Cost-Effectiveness: Slab on grade foundations are generally cost-effective compared to deeper foundation options like basements or crawl spaces. They require less excavation and construction materials, which can be beneficial considering the current increase in construction material costs and labor shortages.
9. Construction Efficiency: Shallow, slab on grade foundations are relatively straightforward to construct, leading to faster construction times and reduced labor requirements.
10. Environmental Considerations: Slab on grade foundations can be a more sustainable option as they minimize the amount of concrete used and reduce the environmental impact of construction.
The Evolution of Shallow Foundations:
Throughout history, the evolution of shallow foundation design in the United States was driven by the influx of settlers and the need for more sophisticated construction practices that date back to the 1600’s. From the early European settlers, to the Industrial Revolution, these events paved the way for a combination of early engineering principles to be derived, advancements in construction materials, and the need to meet changing construction demands. In the mid 1800’s, professional organizations, such as the American Society of Civil Engineers (ASCE), were established. By the late 1800’s, we saw advancements in structural analysis and load calculation methods. Portland cement and reinforced concrete structures were developed during this period, and engineers began incorporating footings and reinforced concrete slabs into building designs. During the 1920’s, a French engineer pioneered the technique of post-tensioning, recognizing the potential benefits of applying compressive forces to concrete structures to counteract tensile stresses, which improves the load-carrying capacity and reduces the risk of cracking and deformation.
After World War II, the United States experienced a boom in construction, where we saw more advancements in the engineering field. The introduction of soil mechanics as a scientific discipline provided a better understanding of soil behavior and improved foundation design methods.
For more than 50 years, our construction methods have not evolved to the degree that other industries have, like airplanes, automobiles, computers, or cell phone. We are currently living in a time where we witnessed digital computers revolutionize our everyday lives, Silicon Valley became the hub for technological advancements, and Quantum computers now hold the potential to bring another major shift in our technology environment. Meanwhile, our construction industry continues to follow the same construction methods, but are we truly designing the most efficient design with the tools and resources that we have available? Research and innovation are the pinnacle of engineering and essential to our growth and evolvement. It is important that we continue to push the boundaries of what is possible, what can be optimized, and ways to improve our methods. The future is now.
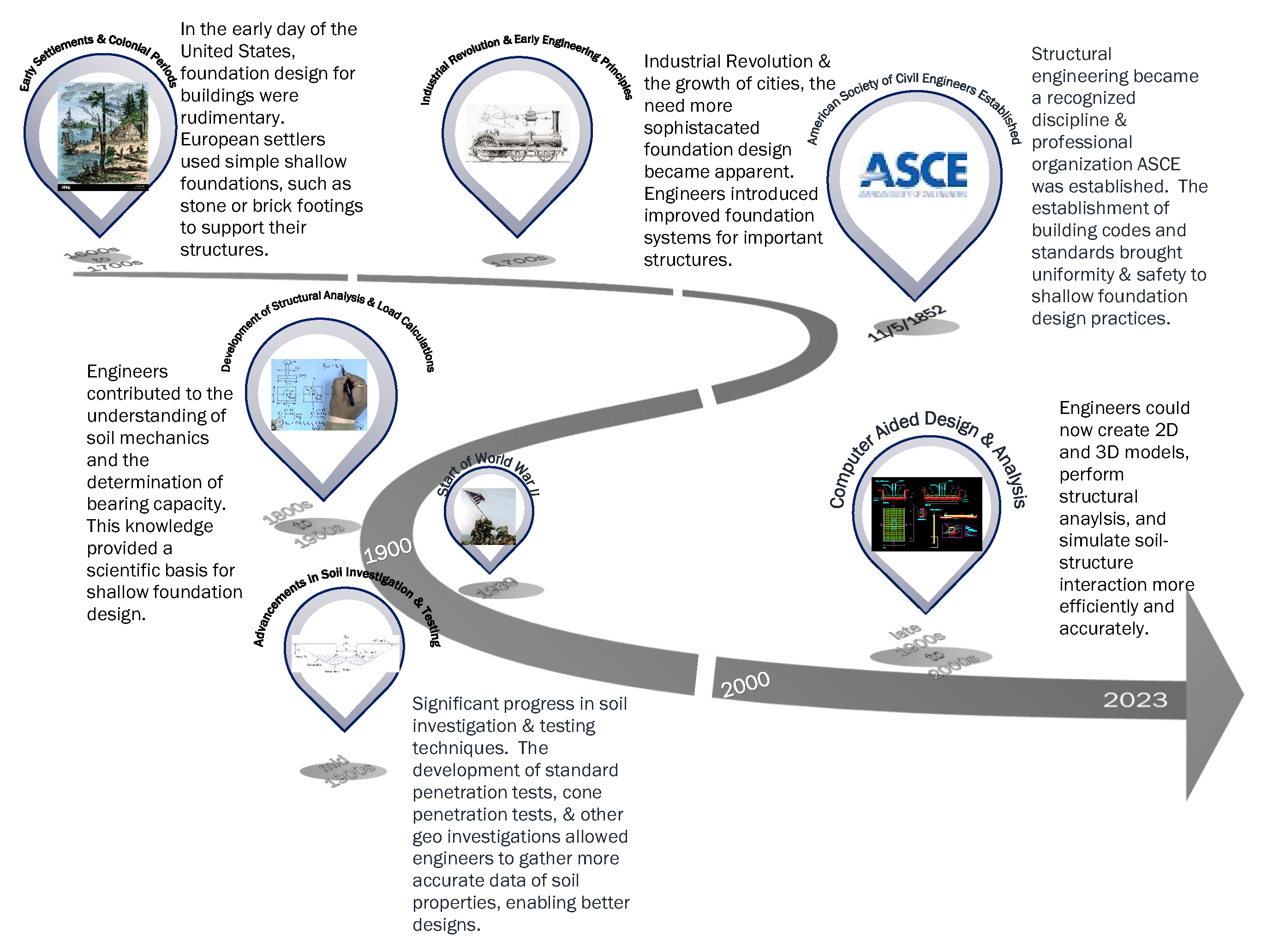
Modern Day
It is important to note that shallow, slab on grade foundations may not be suitable for every building or soil condition type. In areas with poor soil conditions or high water tables, other foundation types like deep foundations or suspended floor systems may be more appropriate. As engineers, our responsibility is to carefully analyze site-specific key factors, as listed in the key objectives, that prompts us to make informed decision regarding the type of foundation design that is best suited for each project.
During the pandemic in 2020, every industry, including Engineering and Construction, had to adjust to new norms. For most of us, we experienced an increased demand for engineered designs of projects while the government offered low-interest loans and fiscal packages. At the same time, we experienced supply chain disruptions that caused an increase in cost of materials, goods and labor. Now that things have settled, and the pandemic was officially declared over in May 2023, we are left with high material costs, a reduction of skilled labor workers, a quarterly increase on the price of concrete, and high interest rates. Additionally, there has been a growing emphasis on green and resilient infrastructure designs, promoting environmentally friendly and adaptable solutions for the future.
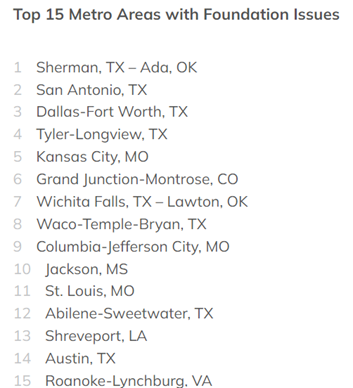
As structural engineers continue to design foundations, we trust that our design recommendations are conservative enough to withstand all the key point considerations in a foundation design, however, San Antonio, TX is currently cited as the 2nd worst US metro for foundation issues, with Dallas, Houston and Austin making the top 15 list in the country, according to Top 15 U.S. Metro Areas with Foundation Issues – Groundworks, as shown in Figure 3. The locations listed with the top foundation issues are also areas with highly expansive clay soils. Which brings up the question, are our foundation designs resilient enough to withstand the soil forces induced by the variation in moisture? Additionally, is our soil-structure interaction accurately representing true environmental conditions?
Wafflemat Introduction
Wafflemat, is a permanent, repurposed plastic, hollow, void form box that displaces concrete to form interior beams around the Wafflemat boxes. The interior beams are created with a series of interlocked square or rectangular voids in a grid pattern within the slab, reducing the amount of concrete used when compared to traditional ribbed or uniform thickness slab. The Wafflemat boxes are placed on top of a level, compacted subgrade in Texas. The foundation also has a trenched exterior beam to mitigate moisture variation. This innovative shallow foundation provides a cost-effective and efficient solution for supporting buildings on expansive or compressible soils. Additionally, the Wafflemat Foundation System can be designed by using either post-tension cables or conventional reinforcement, however, for maximum potential cost savings, we have found post tension foundations to be a more cost effective solution.
Wafflemat: Case Study
In our efforts to validate our enhanced structural performance, we have performed an in depth comparison of a 40 feet by 70 feet foundation as a case-study (Figures 6 and 7). There is currently no industry standard on how to effectively analyze a slab on grade foundation using Finite Element Modeling (FEM), so we created one. We applied the Post Tensioning Institute DC 10.5 (PTI) design approach and started with the traditional foundation design as a benchmark. We analyzed the design using the GTK PTISlab 3.5, primarily because it is offered by the same company that developed the VoFlo software that most geotechnical engineers use to provide their design considerations in their soils report, and performed a direct side by side comparison with the FEM results (Figure 4). We ran three design iterations to check soil bearing pressure, edge lift mode and edge drop (formerly referred to as Center Lift).
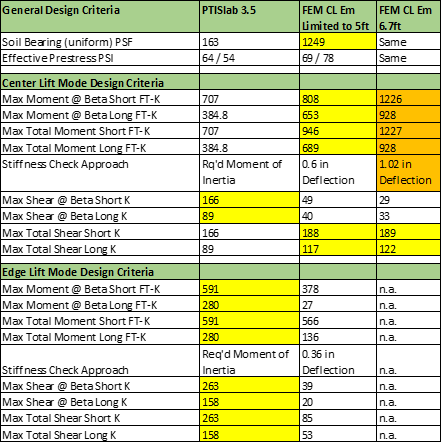
After we compared the traditional foundation design with both the PTI Slab 3.5 software and FEM, we applied the same approach to analyze a Wafflemat Foundation by using the same process of analyzing the bearing pressures, edge lift and edge drop conditions. Our calculations resulted in a reduction of 25% in concrete with a Wafflemat foundation design when compared to a traditional ribbed foundation, a 40% reduction in post tension cables, a 5% increase in the moment of inertia in the short direction, and 40% increase in the moment of inertia in the long direction.
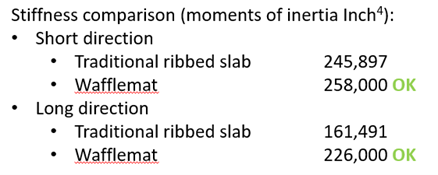
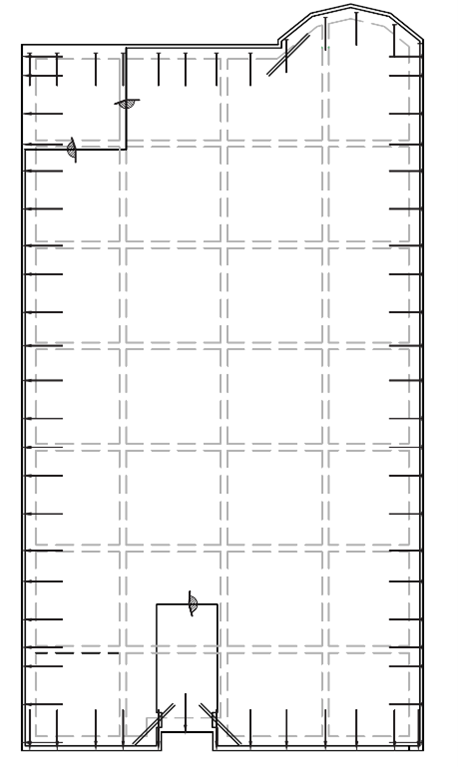
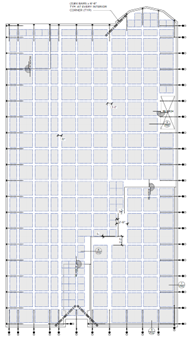
Results and Discussions:
The results of the parametric study proved that the Wafflemat Foundation uses less concrete, less tendons, and offers a stiffer cross section in both directions. Additionally, the prepared subgrade is not disturbed with interior trenching of the slab, allowing for the structural integrity of the soil to remain in tact. It also offers quality control of the ribbed beams by eliminating the possibility of the ribbed beams being overexcavated, as well as ensuring the reinforcing is properly secured at its intended elevation and placement.
The PTI method is an empirical design method that is derived from a 40 feet by 40 feet foundation footprint. The current methodology has a limitation with the geometry of the foundation. The code states that the shape factor is defined as Shape Factor = Perimeter2 / Area. This value should be 24 or less, because of how the equations are written. Foundation designs that exceed a Shape Factor value of 24, are instructed to adjust the building footprint or use finite element analysis to analyze the foundation. Additionally, there is a discontinuity in the long edge, center lift moment equation. The current solution is to revert the em value to 5’, regardless of the Geotechnical report values.
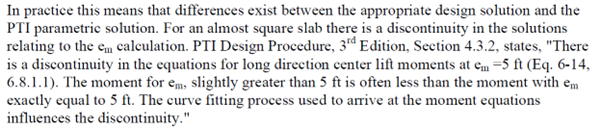
Conclusion:
The utilization of Wafflemat in shallow foundation designs offers a promising and innovative approach to enhance structural performance. Through our evaluation of numerical simulations and case studies, several key findings have emerged.
Key Findings:
- Reduction in material usage – The reduction in slab thickness and overall concrete usage allows for a reduction in carbon dioxide emissions. According to The Architects Newspaper January 2, 2019 edition, concrete production produces eight percent of the world’s carbon dioxide emissions.
- Cost effective – Wafflemat foundations are designed for construction efficiency, they are assembled faster than current construction methods, eliminates the need for interior trenching, allows for an overall reduction in material usage.
- Load distribution capabilities – With a Wafflemat design, only 20 to 25 percent of the interior beams are in contact with soil, allowing for a minimum of eight inches of separation from the bottom of the slab to the building pad, minimizing the application of external soil forces to work against the foundation design. Refer to Figure 9 and 10.
- Reduced settlement – The grid of reinforced concrete beams at 44 inches on center in both directions allows the effective disbursement of loads over a wider area, reducing stress concentrations and mitigating the risk of foundation failures.
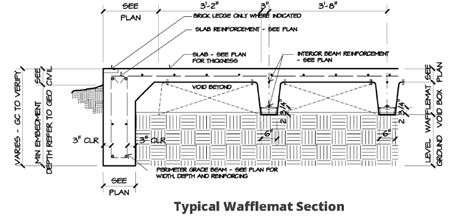
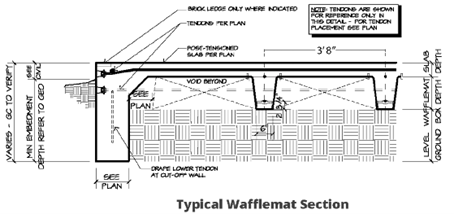
It is important to note that the success of Wafflemat Foundations relies on careful consideration of soil conditions, structural demand requirements, and construction considerations. Adhering to proper design guides and recommendations is crucial to ensure optimal performance and longevity.
Wafflemat technology has demonstrated its ability to enhance structural performance of shallow foundations. By adopting this innovative approach, engineers and designers can create more resilient and economically viable structures. Further research and development are still necessary to explore the full extent of Wafflemat’s capabilities and to redefine methodologies. With continued advancements in this field, Wafflemat will continue its efforts to contribute to the resilient, sustainable, and economically viable structures of the future.
Anna Olveda, MSCE joined Wafflemat as a Sales Engineer in 2022, where she is currently the VP of Engineering. In the past 8 years of her engineering career, she has designed numerous commercial, industrial, multi-family and residential projects across the state of Texas that vary in size and complexity. She received her Bachelor of Science in Civil Engineering with a minor in Mathematics from Texas A&M University in Kingsville, TX (TAMUK) in 2013 and a Master of Science in Civil Engineering (TAMUK) in 2015 with an emphasis in Structural Engineering.
Figures(s) courtesy of: Wafflemat